Scientists currently believe that there are just four fundamental interactions influencing matter and radiation – the electromagnetic, weak nuclear and strong nuclear forces, and gravity. One of science’s great quests is to unify all four into one fundamental force, such as may have existed at the extraordinarily high energy levels of the embryonic Universe. If we were to travel back in time to the very earliest moments after the Big Bang, with the energy levels of the Universe rising as we go, it’s believed that first we would experience the unification of the electromagnetic and weak interactions, then farther back the grand unification of the electroweak force with the strong nuclear force, then finally the superunification of these three with gravity.
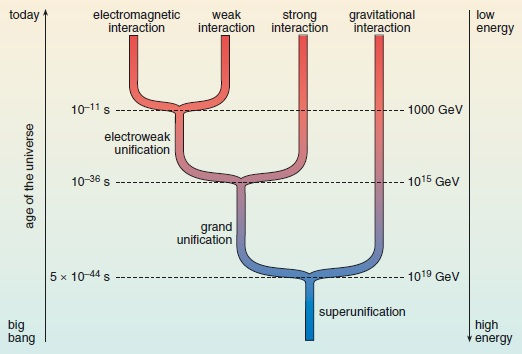
The energy levels of particles in the Universe have slowly declined since the Big Bang
A unified electroweak theory must encompass the three weak quanta, the W and Z bosons, which have mass, and the electromagnetic quantum, the photon, which is massless. Current thinking postulates the existence of four fields, one corresponding to each quantum: three of these fields give mass to the three weak quanta, but the fourth, instead of giving mass to the photon, will manifest as a particle – the much-hypothesised Higgs boson – with a mass energy between 100 and 1000 GeV. In other words, in this energy range the electromagnetic and weak forces will appear to be unified. The chance to prove or disprove electroweak unification is now within our reach, with the Large Hadron Collider at CERN and other similarly high-powered particle-smashers seeking to discover the elusive Higgs boson – or perhaps something more surprising instead!
The next force to be reconciled with the electroweak is the strong nuclear force. According to quantum electrodynamics, the strength of electromagnetic interactions increases slowly with increasing energies; according to quantum chromodynamics, the strength of strong interactions decreases with increasing energies. It is believed that at energies of around 1015 GeV the strengths of the two forces become comparable, and the electromagnetic and strong forces may achieve a grand unification. To achieve this unification another particle, termed the X boson, is needed, and it’s predicted that the X boson would allow quarks to change into leptons, matter to change into antimatter, and vice versa. We have never witnessed an X boson at work, because its activity would be extremely weak at the energies we can currently manufacture on Earth – it would get properly busy only at the tremendous energies around the 1015 GeV mark. These energies are currently beyond our means to recreate – but we may be able to glimpse the exotic processes that are hypothesised to occur in these conditions, such as the decay of protons, because they may occur, very occasionally, at the more mundane energies of the world around us today. Monitoring a huge enough sample of protons, say 1033, for a few years, may allow us to see a few proton decays.

View inside the detector of the Large Hadron Collider, CERN
Even if we are able to unify the electromagnetic, weak and strong forces, one final, perhaps most recalcitrant, force awaits unification – gravity. Gravity is the most difficult of the four forces to interpret at a quantum level, one great challenge being how we can take the fundamental quantum idea of uncertainty – uncertainty of position and movement of subatomic particles – and somehow apply that idea of uncertainty to space and time themselves. A popular current theory – or rather five versions of the same theory! – speculates that particles such as quarks, leptons and photons may exist as ‘strings’ rather than points – strings which exist in ten dimensions of space-time, whose vibration patterns across all these dimensions can provide all the characteristics needed by such particles, such as mass, colour charge and electric charge. The five string theories may be manifestations of a yet deeper theory, termed M-theory, which postulates objects called ‘branes’ – membranes which can exist in up to eleven dimensions. Strings and branes are still only theories: to experimentally probe superunification would require energy of 1019 GeV – termed the Planck energy – and we are a long way from being able to achieve this.
So tools such as the Large Hadron Collider currently give us good hope of proving (or disproving) the existence of the Higgs boson, thereby gaining a much clearer insight into electroweak unification. Understanding grand unification is a tougher goal, though as mentioned above we may be able to gain insights from experiments that don’t require such exotic energies as grand unification itself. And as for superunification, it’s a noble summit for today’s theoreticians – and tomorrow’s experimenters – to seek to scale!
Andrew Murray 2012
Photos courtesy the Open University and Wikipedia Commons
Comments